Oak Ridge 国立研究所による「Roswell金属破片」の分析結果報告書:pdf → テキスト抽出 → 和訳
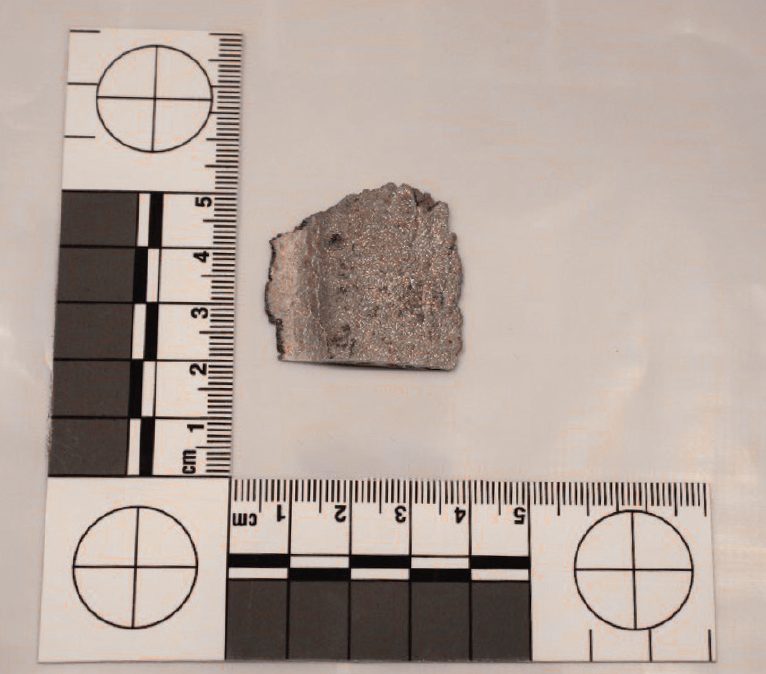
前置き
下の pdf から英文テキストを抽出した。その英文テキストを DeepL(New model)で和訳した。以下では図や写真は無視したので、それらは下の出典にある pdf を参照。
さらに ChatGPT-4o に後述の英文テキスト(参照文献のみ除外)を喰わせて、要約させた。
関連
国立研究所で Roswell の墜落 UFO 由来とされる破片を分析 →普通の地球の物質と判明
要約(by ChatGPT-4o)
序論
All-Domain Anomaly Resolution Office (AARO)は、1947年頃のUAP(未確認異常現象)の墜落から回収されたとされる、マグネシウムと亜鉛からなる層状物質の標本を分析しました。この標本は「慣性質量の減少」(反重力機能)を持つとされ、ビスマスとマグネシウムの層がテラヘルツ波ガイドとして機能する可能性があるとされています。
方法
分析は光学顕微鏡、CTスキャン、走査電子顕微鏡(SEM-EDS)、透過電子顕微鏡(TEM-EDS)を用いて行われ、化学組成や同位体比も質量分析で評価されました。
結果
標本は地球起源であり、ビスマス層はテラヘルツ波ガイドとして機能するための要件を満たしていないことが確認されました。
結論
標本は地球上で製造されたものであり、反重力機能や非地球起源の証拠は見つかりませんでした。標本は機械的および熱的ストレスを受けた形跡がありました。
以上の分析から、標本の科学的および物理的特性が詳細に評価されましたが、非地球起源である可能性は否定されました。
出典(pdf)
予備:本サイトから pdf(9.8MB) を download
はじめに
All-Domain Anomaly Resolution Office (AARO) は、マグネシウムと亜鉛を主成分とし、ビスマスとその他の微量元素の帯状層を持つ積層材料標本の測定を複数回実施しました。この材料標本の起源と目的については、長年にわたり議論されてきた歴史があります。この材料標本は、1947年頃に発生した未確認異常現象(UAP)の墜落現場から回収されたと主張されています。さらに、この試料の物理化学的特性により、この物質は「慣性質量減少」(すなわち、浮遊や反重力機能)が可能であると主張されています。これは、ビスマスとマグネシウムの層がテラヘルツ波の導波路として機能しているためである可能性があります。
これ以前に、米国陸軍戦闘能力開発司令部(DEVCOM)は、この広く議論されている標本に関連する潜在的な破壊的テクノロジーの利用可能性を評価するために、トゥ・ザ・スターズ・アカデミー(TTSA)と共同研究開発協定(CRADA)を締結しました。
2022年に設立されたAAROは、UAP事件の歴史的記録を調査し、その調査結果を公開することを議会から義務付けられています。この標本の長い保管履歴は確認できませんが、標本に対する一般およびメディアの関心の高さを考慮し、科学的方法に従った透明性の高い調査を行うことが求められました。
TTSA-DEVCOM CRADAに続いて、AAROは科学技術パートナーであるオークリッジ国立研究所(ORNL)を確保しました。ORNLは米国エネルギー省が所有する17の国立研究所のひとつであり、80年にわたる世界トップクラスの材料科学の専門知識を活用し、この標本を独自に評価し、徹底的な特性調査を実施しました。
図1. 受領時のバルク標本の様子。一般公開用に内部サンプル追跡情報を編集したステッカー。
材料特性評価の専門家である ORNL は、多様な専門知識を持つスタッフと強力な機器群を擁しており、個々の研究所の能力を超える厳密な科学的調査が可能です。そのため、この試料とその特性について公平な分析を行い、科学的整合性を維持する能力に優れた機関です。
AARO は ORNL に、(1) この試料が地球起源であるかどうか、(2) この試料に含まれるビスマスがテラヘルツ波の導波路として機能する可能性があるかどうかを評価するよう依頼しました。DEVCOM地上車両システムセンターは、2023年2月からORNLに金属試料へのアクセスを提供しました。
ORNLの材料科学分析では、顕微鏡、分光法、分光分析など、複数の方法を用いて試料の構造、化学組成、同位体比を評価しました。その結果は、DEVCOMによる過去の分析結果と一致し、ビスマス層の構造と組成はテラヘルツ波の導波路として必要な要件を満たしていないことを示しました。さらに、すべてのデータは、この物質が地球起源であることを強く裏付けています。
Introduction
The All-Domain Anomaly Resolution Office (AARO) sponsored a series of measurements on a layered material specimen primarily composed of magnesium and zinc, with bands of bismuth and other co-located trace elements. The material specimen, whose origin and purpose are of long and debated history, is claimed to be recovered from an unidentified anomalous phenomenon (UAP) crash in or around 1947. Furthermore, the specimen’s physiochemical properties are claimed to make the material capable of “inertial mass reduction” (i.e., levitation or antigravity functionality), possibly attributable to the material’s bismuth and magnesium layers acting as a terahertz waveguide.
Previously, US Army Combat Capabilities Development Command (DEVCOM) established a Cooperative Research and Development Agreement (CRADA) with To the Stars Academy (TTSA) to evaluate the feasibility of exploiting any potential disruptive technology associated with this widely discussed specimen.
AARO, founded in 2022, is congressionally mandated to explore historical records of UAP incidents and publicly report its findings. Although the long chain of custody for this pecimen cannot be verified, public and media interest in the specimen warranted a transparent investigation that adhered to the scientific method.
Subsequent to the TTSA–DEVCOM CRADA, AARO secured science and technology partner Oak Ridge National Laboratory (ORNL), one of 17 US Department of Energy national aboratories, to independently assess and perform thorough characterization studies on the specimen, leveraging ORNL’s 80-year history of world-leading materials science expertise.
Figure 1. View of the as-received bulk specimen. Sticker containing internal sample tracking information edited out for public release.
ORNL, an expert in materials characterization, has the diverse staff expertise and co-located, powerful instrumentation suites to allow rigorous scientific inquiry beyond the capabilities of most individual laboratories. Therefore, it is a highly qualified institution to maintain scientific integrity in its unbiased analysis of this specimen and its properties.
AARO tasked ORNL with assessing whether (1) the specimen is of terrestrial origin and (2) the bismuth in the specimen could act as a terahertz waveguide. DEVCOM Ground Vehicle System Center provided ORNL access to the metallic specimen—a single parent sample and three previously derived subsamples, all from the same material—beginning in February 2023.
ORNL materials science analyses evaluated the sample’s structure, chemical composition, and isotope ratios via multiple methods, including microscopy, spectroscopy, and spectrometry. Results align with previous DEVCOM analyses, indicating that the structure and composition of the bismuth layers do not meet the requirements necessary to serve as a terahertz waveguide. Furthermore, all data strongly support that the material is terrestrial in origin.
方法
すべての分析および材料の利用は、DEVCOM CRADA を通じて TTSA によって承認および監督され、すべての分析は、ORNL が試料を受け取る前に AARO および DEVCOM によって事前承認されました。
形態および微細構造の特徴は、以下の技術を使用して調査されました。
- 光学顕微鏡:微細構造の特徴を画像化できる標準的な顕微鏡分析。
- コンピュータ断層撮影、別名 CT スキャン: X線画像化技術で、試料を傷つけることなく3D画像を作成し、内部構造の特徴を明らかにします。
- 走査型電子顕微鏡-エネルギー分散型X線分光法(SEM-EDS):2D画像をより高解像度で作成し、微細構造や元素組成の分析を可能にする技術です。
- (走査)透過型電子顕微鏡-エネルギー分散型X線分光法([S]TEM-EDS):高エネルギー(200kVなど) の電子ビームを試料(厚さ200nm未満)の薄膜に通し、結晶構造、結晶粒や特徴の形状、欠陥、元素組成をナノメートルからサブナノメートルの解像度で分析する一連の技術。
バルク化学、元素、同位体組成の分析には、元素を特定し、サンプル内の存在量(ごく微量のものも含む)や同位体組成を明らかにする、広く使用されている分析技術である質量分析技術が使用されました。
サンプルの完全性と機器性能を監視するため、すべての分析においてトレーサブルな品質管理標準物質とメソッドブランクが実行されました。
Methods
All analyses and materials utilization were authorized and overseen by TTSA via the DEVCOM CRADA, and all analyses were preapproved by AARO and DEVCOM before ORNL received the specimen.
Morphology and microstructural characteristics were investigated using the following techniques.
-
Optical microscopy: standard microscope analysis that allows imaging of microstructural features.
-
Computerized tomography, aka CT scan: X-ray imaging procedure that produces a 3D image of a sample without damaging it, revealing interior structural features.
-
Scanning electron microscopy-energy dispersive x-ray spectroscopy (SEM-EDS): technique that produces 2D images at higher resolution to allow analysis of microstructure and elemental makeup.
-
(Scanning) transmission electron microscopy-energy dispersive x-ray spectroscopy ([S]TEM-EDS): a suite of techniques that pass a high-energy (e.g., 200 kV) electron beam through a thin (<200 nm) foil of sample, allowing analysis of crystal structure, grain and feature morphology, and defects, as well as elemental makeup, all with nanometer to subnanometer resolution.
Analyses of bulk chemical, elemental, and isotopic composition used mass spectrometry techniques, a suite of widely used analytical techniques that identify elements, their abundance within a sample (including very trace quantities), and their isotopic composition.
Traceable quality control standards and method blanks were run throughout all analyses to monitor sample integrity and instrument performance.
結果
形態と構造に関するデータは、複数の画像解析手法で一貫しており、この物質は複数の点で融合したり分岐したりする明確な層から構成されていることを示しています。
界面には亀裂やその他の特徴が見られ、ORNLは、この物質はもともと一体のものであったが、熱への曝露や機械的な力によって、おそらく長期間にわたって歪んだものであると結論づけています。図2は、CTを使用して構築された試料の画像です。
表1. 試料(バルクマグネシウム亜鉛マトリックスを除く)の微量元素組成。 すべて、0.1%未満の含有量で「微量」と見なされます。 灰色は誘導結合プラズマ発光分光分析(ICP-OES)の結果、青色は高分解能ICP-MSの結果を示しています。
図2. CTスキャンにより撮影された画像。バルク試料の複数の角度から撮影されており、材料の層状構造(左下)や熱損傷を受けたエッジ(右下)などの特徴が示されている。
Results
Morphology and Structure Data are consistent across multiple imaging approaches, showing that the material consists of distinct layers that merge and diverge at various points throughout the material.
Interfaces showed fractures and other features that ORNL determined are consistent with a material that was originally whole but was strained by heat exposure and mechanical forces, possibly for extended periods. Figure 2 shows images of the specimen constructed using CT.
Table 1. Trace element composition of the specimen (sans the bulk magnesium-zinc matrix), in decreasing order of abundance. All are considered “trace,” at less than 0.1% bundance. Gray reflects results from inductively coupled plasma optical emission spectroscopy (ICP-OES); Blue reflects results from high-resolution ICP-MS.
Figure 2. Images produced via CT showing multiple angles of the bulk specimen, showcasing features including the layer nature of the material (bottom left) and edge with robable heat damage (bottom right).
化学組成
SEM-EDS 分析により、試料に含まれる主な元素はマグネシウムと亜鉛であることが判明しました。マグネシウムは試料全体の約 97.5%、亜鉛は約 2% を占めています。検出された微量元素(表 1)は鉛(Pb)とビスマス(Bi)で(図 3)、鉄(Fe)とマンガン(Mn)もごく微量に含まれていました。最も感度の高い分析手法である誘導結合プラズマ質量分析法(ICP-MS)では、さらに少量のカドミウム(Cd)、タリウム(Tl)、金(Au)、モリブデン(Mo)、スズ(Sn)、バリウム(Ba)の存在が明らかになりました。検出された元素の量が検量線の下限を下回ったり、検出限界を下回ったりした場合は、その元素が製造工程に意図的に添加された可能性が非常に低いと考えられるため、表1には表示されません。
図3: 1つのサブサンプルからの後方散乱電子像(BSE、左上)とエネルギー分散型X線分光法(EDS)マップ(SEMビームエネルギー:30 kV)。推定重量パーセントで表示(微量元素は表示されていないため、合計は100%にならない)。炭素(C)マップは埋め込みエポキシを示している。マグネシウムマトリックス(Mg)がはっきり見え、マトリックスの亀裂も確認できる。亜鉛(Zn)マップでは、亜鉛含有量が高い領域と低い領域が示されています。鉛(Pb)とビスマス(Bi)マップの上部には、同じ位置にある層が示されています。
(図5は、バンド状の元素組成の追加イメージングを示しており、複数のPb-Bi層を示しています。)
Chemical Composition
Analysis using SEM-EDS determined that magnesium and zinc are the primary elements present in the specimen, comprising approximately 97.5% and 2% of the material, respectively. Minor elements detected (Table 1) were lead (Pb) and bismuth (Bi) (Figure 3), with lesser trace amounts of iron (Fe) and manganese (Mn). Inductively coupled plasma mass spectrometry (ICP-MS), the most sensitive analysis technique performed, additionally revealed the presence of small amounts of cadmium (Cd), thallium (Tl), gold (Au), molybdenum (Mo), tin (Sn), and barium (Ba). If a detected element abundance fell beneath the lower bound of the calibration curve or below the method detection limit, then the element is not displayed in Table 1 because that element was extremely unlikely to have been a purposeful addition to the manufacturing process.
Figure 3: Backscattered electron image (BSE, top left) and energy dispersive x-ray spectrometry (EDS) maps from one subsample (SEM beam energy: 30 kV), presented as estimated weight percent (minor elements not shown, so numbers will not total 100%). Carbon (C) map indicates the embedding epoxy. The magnesium matrix (Mg) is clearly visible, along with the cracks in the matrix. The zinc (Zn) map shows regions of higher and lower zinc content. At the top of the lead (Pb) and bismuth (Bi) maps, co-located layers are visible.
(Figure 5 presents additional imaging of the banded element composition, showing multiple Pb-Bi layers.)
結晶構造
TEMにより、試料中のマグネシウムの結晶構造は一般的なマグネシウム合金の構造と一致していることが明らかになりました(図4)。レーザーアブレーションICP-MSにより、亜鉛成分のバンド構造が明らかになり、そのバンドには鉛とビスマスが層状に(ほぼ1:1の割合で)並んでいることが分かりました。ビスマスが豊富な部分は明確な結晶構造を持たず、非晶質マトリックスの中に非常に微細な結晶ポケットが密集しているように見えました(図4)。 単層で純粋な単結晶ビスマスは、電気やエネルギーフィールド(この場合、テラヘルツ波(マイクロスケールの波長を持つ電磁波))を乱したり、方向付けたりする物質である導波路として機能する能力を持つと仮定されています。試料へのダメージ(熱ストレスの疑いを含む)により、試料の元の構造を明確に述べることは不可能ですが、現在の試料の検査層におけるビスマスの非晶質およびナノ結晶の外観は、おそらくこの材料内に純粋な結晶質のビスマス層が存在しなかったことを示しています。 さらに、このような理論上のビスマスベースの導波路の構造は、異なる誘電率を持つ物質の間に単層で存在することが必要となります。しかし、物質全体に複数のビスマス層が存在しても、この導波路の機能性を実現したり向上させたりできるとは想定されていません。したがって、試料内の不純物ビスマスが層状構造になっているため、それが導波路として機能しない可能性が高い(図5)。
最後に、ビスマスの仮説上の用途に基づいて考えると、ビスマスが導波路として機能するために必要な誘電特性は、試料内のビスマスが鉛と混在しているため、この材料では損なわれていると考えられる(図3および5)。これらの調査結果に基づき、ORNLは、この物質がビスマスベースのテラヘルツ波ガイドとして機能した可能性は極めて低いと結論付けた。
図4:(a)試料のバルクから単結晶領域を切り出したTEM写真。この解像度では構造欠陥が観察できる(この図は、図3に示された領域のわずか2~3ピクセルに相当する領域を示している)。下部の縦線は、集束イオンビームによる試料作成の痕跡です。 (b) (a) の領域から得られた選択領域電子回折パターン (SAEDP)。標準的な Mg 構造にインデックス付けされています。 (c) 低倍率モンタージュ TEM 顕微鏡写真。ビスマスが豊富な層 (中央の暗い帯) が密集している領域。 (d) ビスマスが豊富な層からの SAEDP。ビスマスが豊富な領域からの電子回折パターン(画像処理γ=3.0)が白いリングで示されています。比較のために、ビスマス(Bi、黄色)と Bi2O3(赤)の計算されたリングパタ ーンが示されています。Biの方がわずかに一致度が高いです。 この回折パターンは、ビスマス層がナノ結晶で欠陥が多いことを示しています。
図5. レーザーアブレーションICP-MS元素マップ。(上)鉛(緑)、ビスマス(青)、亜鉛(赤)の3つの主要微量元素の分布。色の混ざり具合で重なり具合を表しており、青緑色は鉛とビスマスがほぼ1:1の割合であることを示しています。(下)ビスマス濃度の元素マップ(黄色が濃いとビスマスが多く、紫色がかっているとビスマスが少ない)。ビスマスは上部に最も多く存在していますが、多くの層で繰り返して見られます。
Crystalline Structures
TEM revealed that the crystalline structure of magnesium in the specimen was consistent with common magnesium alloy structures (Figure 4). Laser ablation ICP-MS revealed banding of the zinc components, along with layered colocation of lead and bismuth (in an approximate 1:1 ratio) in the bands. The bismuth-rich portions of the specimen lacked a clear crystalline structure, instead appearing to consist of highly nanocrystalline pockets in an otherwise amorphous matrix (Figure 4). Pure single-crystalline bismuth in a single thin layer has been postulated to have the ability to function as a waveguide, a material that can disrupt or direct an electric or energy field—in this case, terahertz waves (electromagnetic waves with microscale wavelengths). Although the damage to the specimen (including suspected heat stress) precludes a definitive statement describing the specimen’s original structure, the amorphous and nanocrystalline appearance of the bismuth in the examined layers of the current specimen likely indicates that a pure crystalline layer of bismuth was never present within the material. Moreover, the postulated structure of such a theoretical bismuth-based waveguide requires it to be in a single layer between a material possessing a different dielectric constant. However, multiple bismuth layers throughout a material have not been postulated to be capable of achieving or improving this waveguide functionality—in fact, multiple layers could instead interfere with such functionality. Thus, the layered nature of the impure bismuth within the specimen likely precludes it from acting as a waveguide (Figure 5).
Finally, based on the postulated hypothetical uses of bismuth, the dielectric properties necessary for bismuth to function as a waveguide would have been disrupted in this material because the bismuth in the specimen is colocated and mixed with lead (Figures 3 and 5). Based on these findings ORNL determined that this material is highly unlikely to have ever functioned as a bismuth-based terahertz waveguide.
Figure 4: (a) TEM micrograph of a single-crystalline region from the bulk of the sample; structural defects are visible at this level of resolution (this figure is showing an area that represents just 2 to 3 pixels of the area shown in Figure 3). The vertical lines at the bottom are a focused ion beam preparation artifact. (b) Selected area electron diffraction pattern (SAEDP) from the region in (a), indexed to the standard Mg structure. (c) A low-magnification montage TEM micrograph of an area showing a dense bismuth-rich layer (dark central band). (d) A SAEDP from a bismuth-rich band. The electron diffraction pattern (with image processing γ=3.0) from the bismuth-rich region is shown in the white rings. Both bismuth (Bi, yellow) and Bi2O3 (red) calculated ring patterns are shown for comparison; Bi is a slightly better match. The diffraction indicates that the bismuth layer is nanocrystalline and highly defective.
Figure 5. Laser ablation ICP-MS elemental maps. (Top) Colocation of lead (green), bismuth (blue), and zinc (red), the three primary minor elements in the material. Blending of colors indicates co-location: teal indicates the nearly 1:1 ratio of lead to bismuth. (Bottom) Elemental map of bismuth concentration (hot [yellow] = more, cool [purple] = less). Bismuth is most concentrated at the top but is present in many repeating layers.
同位体分析
多チャンネル ICP-MS 分析により、この試料のマグネシウムと鉛の同位体組成は、地球上で製造・使用されている他の物質と一致することが明らかになりました(図 6 および 7)。同位体は、質量が異なる同じ元素のさまざまな形態であり、その割合によって同位体が含まれる物質の化学的性質が変化し、その物質の歴史に関する情報が明らかになります。すべての元素には同位体があり、さまざまな同位体の量の比率は同位体シグネチャと呼ばれ、化学分析における指紋のようなものです。
試料のマグネシウム同位体シグネチャは分画されていますが(おそらく、この物質が受けたと思われる機械的および熱的歪みのため)、通常の地球組成の範囲内にあり、分画の予想されるトレンドラインに正確に一致しています(図6)。それぞれの恒星系は、その恒星形成領域から受け継いだマグネシウム同位体組成を持っています。図6は、太陽系内で生成されたさまざまな物質のマグネシウム同位体組成を示しています。下部の図表の直線は、質量に基づく同位体シフトの種類を定義する動的および平衡質量分 画傾向線です。分画は化学反応や物理的プロセス(製造や採掘など)によって起こり、天然素材や人工素材、それらの構成要素の寿命の間は通常起こります。図6の素材(試料を含む)は分画トレンドライン上またはその付近に位置しており、その出発組成はかつて同じであり、分画の結果、系統的に変化したことを強く示しています。もしその物質が太陽系外から来たものである場合、マグネシウム同位体比は、図6の上部グラフのほぼどの位置にもプロットされる可能性があります。一方、この試料のデータは、太陽系特有の既知の組成に対する予想される分画傾向線内に正確にプロットされています。
より単純に言えば、この試料の鉛同位体組成は、地球や地球上の物質に自然界に存在する「一般的な鉛」の組成と完全に一致しています(図7)。これは月の物質とも明確に区別されており、この物質が地球で生成された可能性が非常に高いことを示しています。
図6:未知物質のマグネシウム同位体系統を含む芸術的表現。特に、地球上の物質、地球外の物質、太陽系外の物質との相対的な関係を示しています。この図は、既知の標準物質(DSM-3)に対する26Mg/24Mgおよび25Mg/24Mg同位体の差を、1000分の1(‰)単位で示したδ26Mg′とδ25Mg′の関係をプロットしたものです。この計算は、ICP-MS分析に固有の既知の機器質量偏り効果を補正したもので、文献でしばしば示されるマグネシウムデータの表示方法と一致しています。(上)異なるタイプの星から得られるマグネシウム同位体組成の極値。 (下)太陽系物質と問題の物質のマグネシウム同位体組成の拡大図。 不確実性エンベロープは含まれていませんが、不確実性を含めてプロットすると、データポイントは動的分画線と平衡分画線に重なります。注:菱形で示されたデータポイントは、完全な情報として含めたもので、古い分析資料から抽出されたものです。これらのデータの正確さや正確性を直接検証することはできません。デルタ値はDSM-3を基準に計算され、系統的なオフセット補正係数が適用されています。 これらのポイントのエラーバーは±2‰の不確かさです。
サンプルA
銀貨 工業用鉛 鉛鉱石 大気浮遊粒子状物質 雲母 隕石 トロイライト 火星
SRM-982 測定値 SRM 982 SRM 981 SRM 983 太平洋硫化物 月面ガラス ガソリン
図7. 未知物質の鉛(Pb)同位体系統。206Pb/204Pb 対 207Pb/206Pb で、他の地球物質および非地球物質と比較した。このプロットには、3つの末端成分組成がある。(1) 太陽系内の鉛の初期組成である原始鉛、(2) 天然ウランの崩壊によって生成される純粋な放射性鉛、(3) 繰り返し分析によって定義される地球上の鉛または「一般的な鉛」。この試料は、地球上の鉛組成の領域に正確にプロットされる鉛同位体組成を持っています。SRM は標準参照物質を意味し、その組成や特性が十分に把握されているため、機器の校正に一般的に使用される物質です。 米国商務省標準技術局が測定および認証しています。
Isotope Analysis
Multicollector ICP-MS analysis showed that the specimen’s magnesium and lead isotope composition is consistent with other materials manufactured and used terrestrially (Figures 6 and 7). Isotopes are varying forms of the same element with differing mass, and their proportions affect the chemical properties of and reveal information about the history of the material within which the isotopes are found. All elements have isotopes, and the ratio between the amounts of various isotopes is called the isotopic signature, which is akin to a fingerprint in chemical analyses.
The magnesium isotopic signature of the specimen is fractionated (possibly owing to the mechanical and heat strain that the material appears to have undergone) but falls within normal terrestrial compositions and precisely within the expected trendlines of fractionation (Figure 6). Each star system has a magnesium isotopic composition that was inherited from its local star-forming region. Figure 6 shows the magnesium isotopic signature of various materials originating within our solar system. The straight lines in the bottom graphic representation are the kinetic and equilibrium mass fractionation trendlines, which define the types of isotopic shifts incurred on the basis of mass. Fractionation occurs due to chemical reactions and physical processes (e.g., manufacturing and mining) and is normal during the lifespan of natural and manufactured materials and their components. The materials in Figure 6—including the specimen—fall on or near the fractionation trendlines, strongly indicating that their starting compositions were once the same and have been systematically changed as a result of mass fractionation. If a material originated outside our solar system, its magnesium isotopic signature could plot nearly anywhere in the top graphic representation of Figure 6—ins tead, the specimen’s data plots it precisely within the expected fractionation trendlines for known compositions specific to our solar system.
Less complexly, the lead isotopic signature of this specimen is fully consistent with “common lead” compositions that exist naturally on Earth and within terrestrial materials (Figure 7), distinctly separate from even lunar materials, indicating it is extremely likely that the material originated on Earth.
Figure 6: Artistic representation including the magnesium isotope systematics of the unknown material, specifically shown relative to other terrestrial, non-terrestrial, and extrasolar materials. The figures plot the δ26Mg′ vs. the δ25Mg′, which represent the differences in 26Mg/24Mg and 25Mg/24Mg isotopes relative to a known standard (DSM-3) in parts per thousand (‰) notation. This calculation adjusts for known instrumental mass bias effects inherent in ICP-MS analyses and is consistent with how magnesium data in literature are often presented. (Top) The extreme magnesium isotope compositions possible from different star types. (Bottom) A zoomed-in view of the magnesium isotope compositions of local solar system materials and the material in question. Uncertainty envelopes are not included, but when plotted with their uncertainties, data points overlap the kinetic and equilibrium fractionation lines. (Note: Data points shown with a diamond shape, included for completeness, were extracted from an older analysis source; we cannot directly verify the precision or correctness of these data. The delta values were calculated relative to DSM-3, and a systematic offset correction factor was applied; error bars for these points reach ± 2 ‰ uncertainty.)
Sample A
Silver Coins Industrial Pb Galena Airborne Particulate Matter Feldspars Meteorite Troilite Mars
SRM-982 measured SRM 982 SRM 981 SRM 983 Pacific Ocean Sulfides Lunar Glasses Gasoline
Figure 7. Lead (Pb) isotope systematics of the unknown material, shown relative to other terrestrial and non-terrestrial materials in 206Pb/204Pb vs. 207Pb/206Pb. This plot has three end-member compositions: (1) primordial lead, which is the starting composition of the lead in the solar system; (2) pure radiogenic lead from the decay of naturally occurring uranium; and (3) terrestrial lead or “common lead,” which is defined by repeat analyses. The specimen has a lead isotopic composition that plots precisely in the field of terrestrial lead compositions. SRM stands for standard reference material, materials typically used to perform instrument calibrations due to their well-characterized composition or properties, as measured and certified by the U.S. Department of Commerce’s National Institute for Standards and Technology.
結論
AAROは、この歴史的な標本が地球外起源であり、ビスマスベースのテラヘルツ波ガイドとして機能できるとする主張を立証または反証するために必要な要件を、ORNLが独自に評価することを確保しました。この標本の起源、保管状況、最終的な用途は依然として不明ですが、化学組成と構造、特性に関する最新の堅牢な分析では、その起源が地球外であることを示す結果は得られていません。また、調査対象物質がテラヘルツ波ガイドとして機能する可能性のある純粋な単結晶ビスマス層を有していたことを示すデータもありません。この物質が過去に意図的または実際に使用されたかどうかは不明ですが、ORNLは、すべてのデータが、この物質が今日の基準では珍しい元素の混合を用いて製造されたものであることを示しているものの、機械的および熱的ストレス要因による損傷を受けたものであるという高い確信を持っています。
Conclusion
AARO secured ORNL to independently assess the requirements necessary to confirm or contest public claims that this historical specimen is of non-terrestrial origin and that it is capable of functioning as a bismuth-based terahertz waveguide. Although the origin, chain of custody, and ultimate purpose of this specimen remain unclear, a modern and robust analysis of its chemical and structural composition and properties does not indicate that its origin is non-terrestrial, nor do the data indicate that the material examined ever had the pure single-crystalline bismuth layer that could possibly have acted as a terahertz waveguide. The intended or actual past use of the material remains undetermined, but ORNL has a high level of confidence that all data indicate the material was manufactured terrestrially—albeit using an uncommon mixture of elements by today’s standards—and then incurred damage caused by mechanical and heat stressors.
References
-
Akram, W.; Schönbächler, M., Zirconium isotope constraints on the composition of Theia and current Moonforming theories. Earth Planet Sc Lett 2016, 449, 302-310.
-
Albarede, F.; Blichert-Toft, J.; Gentelli, L.; Milot, J.; Vaxevanopoulos, M.; Klein, S.; Westner, K.; Birch, T.; Davis, G.; de Callataÿ, F., A miner’s perspective on Pb isotope provenances in the Western and Central Mediterranean. J Archaeol Sci 2020, 121.
-
Blichert-Toft, J.; Zanda, B.; Ebel, D. S.; Albarède, F., The Solar System primordial lead. Earth Planet Sc Lett 2010, 300 (1-2), 152-163.
-
Budde, G.; Tissot, F. L. H.; Kleine, T.; Marquez, R. T., Spurious molybdenum isotope anomalies resulting from non-exponential mass fractionation. Geochemistry-Germany 2023, 83 (3).
-
Catanzaro, E. J.; Murphy, T. J.; Shields, W. R.; Garner, E. L., Absolute Isotopic Abundance Ratios of Common Equal-Atom and Radiogenic Lead Isotopic Standards. J Res Nbs a Phys Ch 1968, A 72 (3), 261-+.
-
Chakrabarti, R.; Jacobsen, S. B., The isotopic composition of magnesium in the inner Solar System. Earth Planet Sc Lett 2010, 293 (3-4), 349-358.
-
Connelly, J. N.; Bizzarro, M.; Thrane, K.; Baker, J. A., The pb-pb age of angrite SAH99555 revisited. Geochim Cosmochim Ac 2008, 72 (19), 4813-4824.
-
de Vega, C. G.; Chernonozhkin, S. M.; Grigoryan, R.; Costas-Rodríguez, M.; Vanhaecke, F., Characterization of the new isotopic reference materials IRMM-524A and ERM-AE143 for Fe and Mg isotopic analysis of geological and biological samples. J Anal Atom Spectrom 2020, 35 (11), 2517-2529.
-
Fouquet, Y.; Marcoux, E., Lead-Isotope Systematics in Pacific Hydrothermal Sulfide Deposits. J Geophys ResSol Ea 1995, 100 (B4), 6025-6040.
-
Gyngard, K. M. H. a. F., The Presolar Grain Database. In 40th Lunar and Planetary Science Conference, 2009.
-
Higgins, J. A.; Schrag, D. P., Records of Neogene seawater chemistry and diagenesis in deep-sea carbonate sediments and pore fluids. Earth Planet Sc Lett 2012, 357, 386-396.
-
Hoppe, P.; Leitner, J.; Kodolányi, J.; Vollmer, C., Isotope Systematics of Presolar Silicate Grains: New Insights from Magnesium and Silicon. Astrophys J 2021, 913 (1).
-
Hulston, J. R.; Thode, H. G., Variations in S33 S34 and S36 Contents of Meteorites and Their Relation to Chemical and Nuclear Effects. J Geophys Res 1965, 70 (14), 3475-+.
-
Kammerer, C. C.; Kulkarni, N. S.; Warmack, R. J.; Sohn, Y. H., Interdiffusion and impurity diffusion in polycrystalline Mg solid solution with Al or Zn. J Alloy Compd 2014, 617, 968-974.
-
Karl K. Turekian, H. D. H., Presolar Grains. In Treatise on Geochemistry, 2 ed.; 2013.
-
Kodolányi, J.; Hoppe, P.; Gröner, E.; Pauly, C.; Mücklich, F., The Mg isotope composition of presolar silicate grains from red giant stars. Geochim Cosmochim Ac 2014, 140, 577-605.
-
Leitner, J.; Hoppe, P., A new population of dust from stellar explosions among meteoritic stardust. Nat Astron 2019, 3 (8), 725-729.
-
Leonid V. Alekseyev, V. A. P., and Evgenii E. Narimanov, Homogeneous Hyperbolic Systems for Terahertz and Far-Infrared Frequencies. Advances in OptoElectronics 2012, (Modern Trends in Metamaterial Applications), 6.
-
Liu, N.; Dauphas, N.; Cristallo, S.; Palmerini, S.; Busso, M., Oxygen and aluminum-magnesium isotopic systematics of presolar nanospinel grains from CI chondrite Orgueil. Geochim Cosmochim Ac 2022, 319, 296317.
-
Lubecke, V. M.; Mizuno, K.; Rebeiz, G. M., Micromachining for terahertz applications. LEEE T Microw Theory 1998, 46 (11), 1821-1831.
-
Monna, F.; Lancelot, J.; Croudace, I. W.; Cundy, A. B.; Lewis, J. T., Pb isotopic composition of airborne particulate material from France and the southern United Kingdom: Implications for Pb pollution sources in urban areas. Environ Sci Technol 1997, 31 (8), 2277-2286.
-
Moriwaki, R.; Usui, T.; Tobita, M.; Yokoyama, T., Geochemically heterogeneous Martian mantle inferred from Pb isotope systematics of depleted shergottites. Geochim Cosmochim Ac 2020, 274, 157-171.
-
Nemchin, A. A.; Whitehouse, M. J.; Grange, M. L.; Muhling, J. R., On the elusive isotopic composition of lunar Pb. Geochim Cosmochim Ac 2011, 75 (10), 2940-2964.
-
Nguyen, A. N.; Messenger, S., Resolving the Stellar Sources of Isotopically Rare Presolar Silicate Grains through Mg and Fe Isotopic Analyses. Astrophys J 2014, 784 (2).
-
Patterson, C., Age of Meteorites and the Earth. Geochim Cosmochim Ac 1956, 10 (4), 230-237.
-
Rutledge, D. B.; Schwarz, S. E.; Hwang, T. L.; Angelakos, D. J.; Mei, K. K.; Yokota, S., Antennas and WaveGuides for Far-Infrared Integrated-Circuits. LEEE J Quantum Elect 1980, 16 (5), 508-516.
-
Song, Q.; Chen, H.; Zhang, M.; Li, L.; Yang, J. B.; Yan, P. G., Broadband electrically controlled bismuth nanofilm THz modulator. Apl Photonics 2021, 6 (5). 28. Stacey, J. S.; Kramers, J. D., Approximation of Terrestrial Lead Isotope Evolution by a 2-Stage Model. Earth Planet Sc Lett 1975, 26 (2), 207-221.
-
Steele, R. C. J.; Elliott, T.; Coath, C. D.; Regelous, M., Confirmation of mass-independent Ni isotopic variability in iron meteorites. Geochim Cosmochim Ac 2011, 75 (24), 7906-7925.
-
Sturrock, P., Composition analysis of the Brazil magnesium. Journal of Scientific Exploration 2001, 15 (1), 6995.
-
Tatsumoto, M.; Knight, R. J.; Allegre, C. J., Time Differences in Formation of Meteorites as Determined from Ratio of Pb-207 to Pb-206. Science 1973, 180 (4092), 1279-1283.
-
Teng, F. Z., Magnesium Isotope Geochemistry. Rev Mineral Geochem 2017, 82, 219-287.
-
Timmes, F. X.; Woosley, S. E.; Weaver, T. A., Galactic Chemical Evolution - Hydrogen through Zinc. Astrophys J Suppl S 1995, 98 (2), 617-658.
-
Vermeesch, P., IsoplotR: A free and open toolbox for geochronology. Geosci Front 2018, 9 (5), 1479-1493.
-
Wakaki, S.; Tanaka, T., Stable isotope analysis of Nd by double spike thermal ionization mass spectrometry. Int J Mass Spectrom 2012, 323, 45-54.
-
Wang, T.; Schwarz, S. E., Design of Dielectric Ridge Waveguides for Millimeter-Wave Integrated-Circuits. LEEE T Microw Theory 1983, 31 (2), 128-134.
-
White, W. M., Chapter 8: Stable Isotope Theory. In Isotope Geochemistry, 2014; p 720.
-
Wombacher, F.; Eisenhauer, A.; Heuser, A.; Weyer, S., Separation of Mg, Ca and Fe from geological reference materials for stable isotope ratio analyses by MC-ICP-MS and double-spike TIMS. J Anal Atom Spectrom 2009, 24 (5), 627-636.
-
Young, E. D.; Galy, A., The isotope geochemistry and cosmochemistry of magnesium. Geochemistry of NonTraditional Stable Isotopes 2004, 55, 197-230.
-
Young, E. D.; Galy, A.; Nagahara, H., Kinetic and equilibrium mass-dependent isotope fractionation laws in nature and their geochemical and cosmochemical significance. Geochim Cosmochim Ac 2002, 66 (6), 10951104.
(2024-07-21)